Energy Impact Partners: A primer on the next three decades of the energy transition - Part 1.
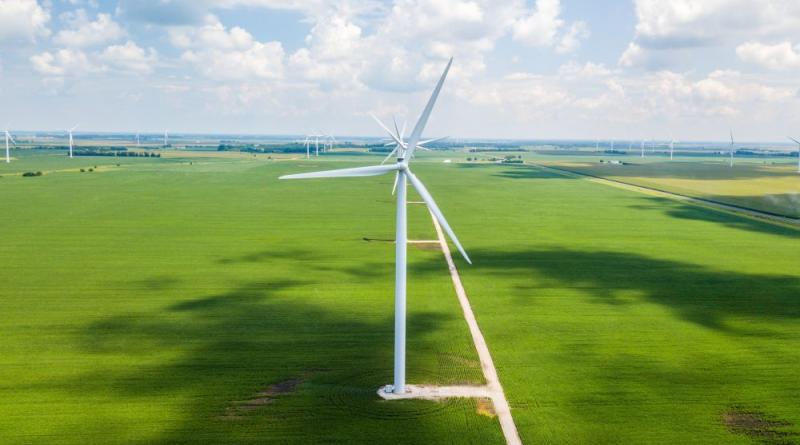
Energy Impact Partners research lead, Andy Lubershane, releases a five-part series examining technologies needed to fully decarbonize the energy system, relying on as few technology “moonshots” as possible.
This article is one of a five-part series examining the technology we’ll need to fully decarbonize our energy system, relying on as few technology “moonshots” as possible.
Part I: The Electric Future We Need
A little historical context
Put yourself in your own shoes, a little over a decade ago, in 2009. At that time, the history of wind and solar growth in the United States looked like this:
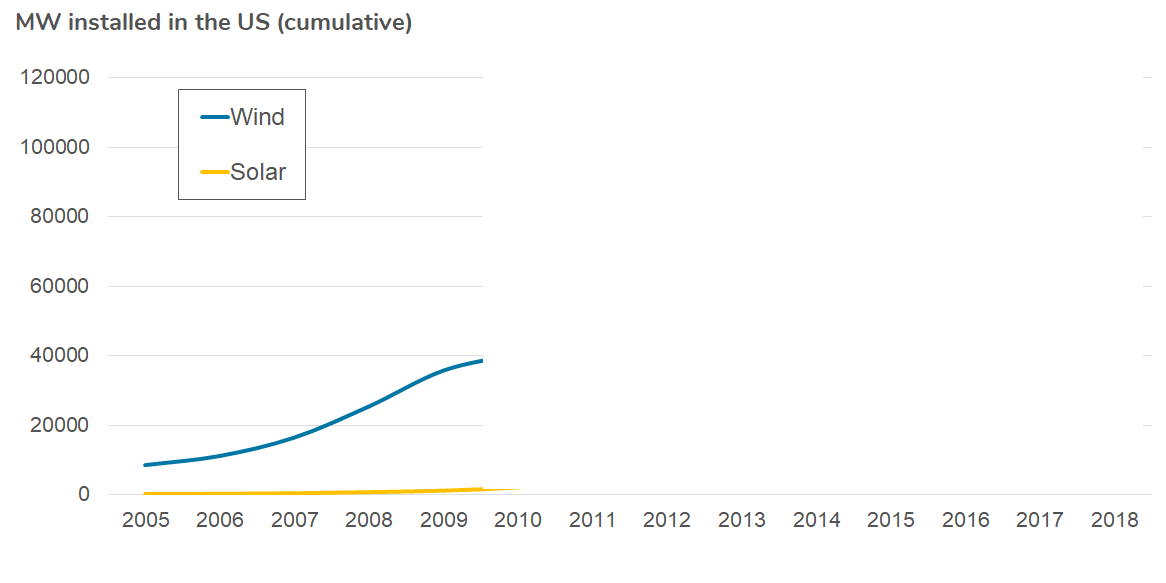
The modern wind energy industry was just beginning to gain traction. European utilities like Iberdrola and EDP had successfully pioneered a new generation of wind turbines, and rising natural gas prices had convinced US utilities to give wind a chance as a hedge against fuel price spikes. The Europeans saw an opportunity to enter the US market by acquiring scrappy, local wind project developers; and a handful of US utilities decided to compete in this emerging “renewable independent power producer” business. In short: the industry was beginning to look like an industry.
Things were looking up for wind, as more and more states established ‘renewable portfolio standards’ which required utilities to source up to 20% of their customers’ electricity demand from renewables. At the time, 20% was considered a wild-eyed target, but the federal government helped take the edge off by offering a healthy tax credit of $23 per megawatt-hour of energy.
Meanwhile, solar still appeared to be more of a tinker-toy for wealthy homeowners, who could afford to pay a premium for rooftop photovoltaics. Costs for the first larger-scale “solar farms” in California exceeded $150 per megawatt-hour, which was a non-starter in the rest of the country.
Back in 2009, imagine if you’d known the following would occur over the course of the next decade:
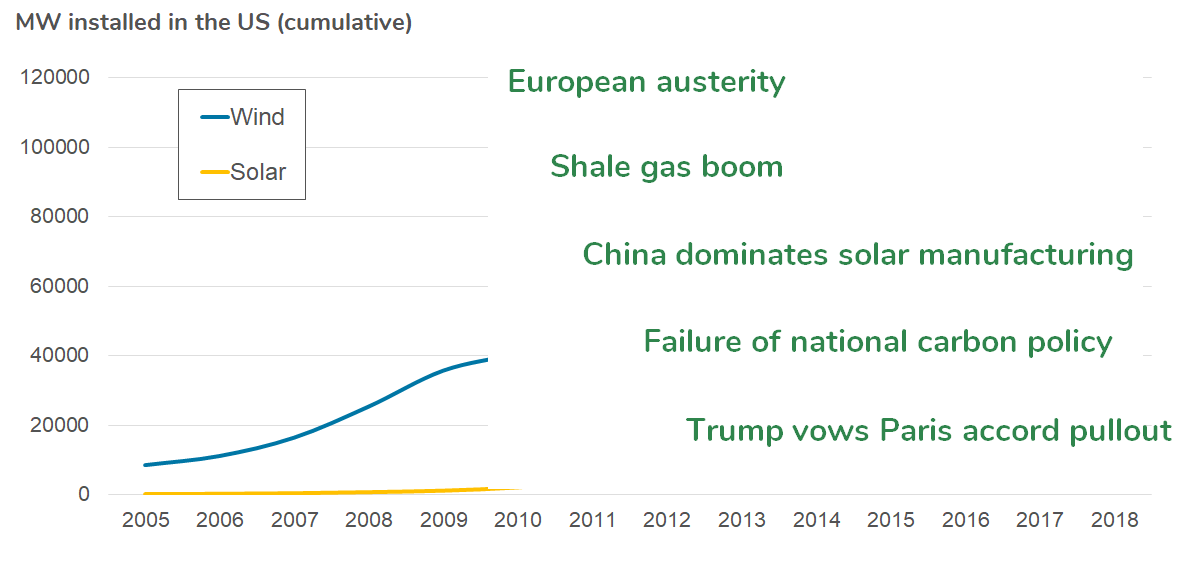
Europe — the beating heart of the renewables market — would enter an extended period of fiscal austerity and pull back from renewable energy subsidies. A revolution in hydraulic fracturing (“fracking”) technology would drive the price of natural gas down from more than $8 per million BTUs to less than $3. The nascent US solar industry — a talking point for politicians aiming to boost solar’s prospects — would be crushed by the entry of Chinese manufacturers. The Waxman-Markey climate bill would fail, as would all future federal carbon policy efforts. And lastly, in 2016, Donald Trump would win the presidency with a vow to pull out of the Paris Climate accord.
From this standpoint in 2009, you’d have been justified in expressing considerable skepticism regarding renewables’ growth prospects. But, you’d have been wrong. Here’s what actually happened:
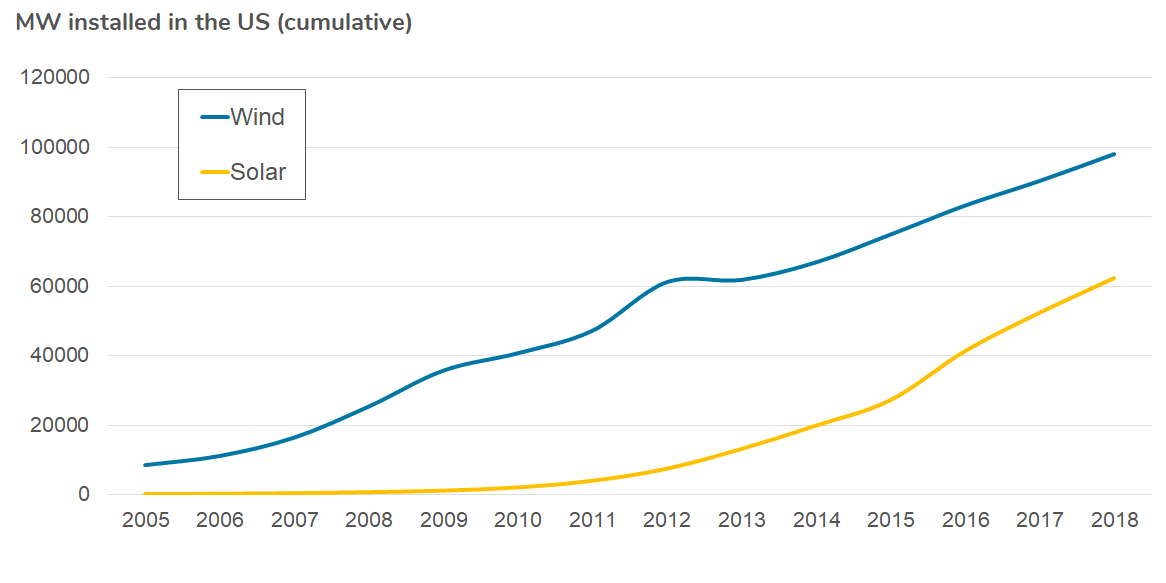
Sometimes history is surprising, even in hindsight. I’d chalk up this surprise to two factors: technology, and policy.
(Full credit to my former colleague Alex Klein for this historical framing.)
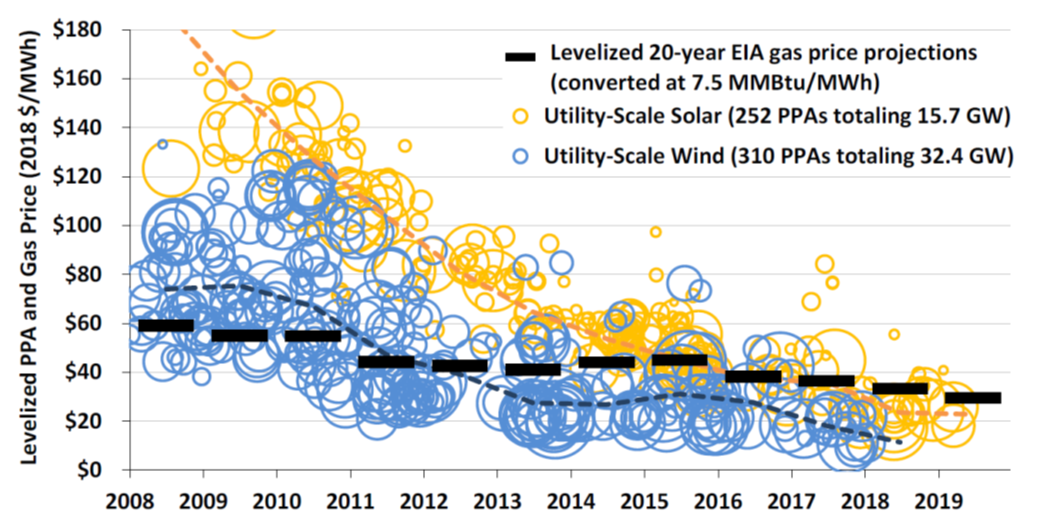
On the technology front, renewables made tremendous strides. Given the support of those (stubbornly persistent) federal tax credits, they kept on competing with natural gas despite the fracking boom. China’s swift domination of solar manufacturing was a temporary political sticking point for US clean energy proponents, but it was also a godsend for the solar cost curve; as the price of solar energy fell by about two thirds in five years. Even wind technology, which was already considered to be relatively “mature” by the early 2010s, continued to beat expectations as blades grew longer, towers taller, and control systems more sophisticated.
On the political front, the headline failure of carbon policy was certainly a disappointment for climate activists. Below the fold, however, a handful of much more important policies went to work. Federal tax credits were twice renewed (albeit at the last minute) with bipartisan backing. Support for renewables has consistently transcended conventional partisan politics. State-level renewable portfolio standard policies held fast, and many have been expanded over time. Today, there are a handful of states with mandatory mid-century targets of 100% clean or renewable energy — a potent rebuke to the skeptics of 20% renewable mandates just a decade ago. More states appear poised to pass similar legislation in the years to come.
In short: the renewable energy industry performed exactly as the world needed it to perform — maturing from infancy, through childhood, into adolescence. Wind and solar power are now reasonably competitive in many regions with the marginal cost of the fossil fuel generation that they displace.
But, we all know that adolescence can be a rocky period. In order to avoid the risk of worst-case climate change scenarios, the world desperately needs renewables to keep maturing. By 2050, at the latest, they will very likely need to be the principal source of energy for human civilization. Wind and solar energy will need to fuel all of the activities for which we currently use electricity, plus many of the activities for which we burn fossil fuel directly — e.g. transport, space heating, and industrial processes.
(Sidenote: There are a handful of plausible scenarios in which we don’t end up relying on renewables as our primary clean energy source by mid-century. One I’ll call “Carbon Disaster”, and the other “Savior Tech”. “Carbon Disaster” doesn’t merit much additional commentary, since others have done such a fine job of describing it. “Savior Tech”, in my mind, includes things like small, modular nuclear reactors; and carbon capture & storage. Research and development in these areas is worth pursuing — aggressively, in order to maximize our chances of avoiding “Carbon Disaster” — but for a number of reasons I believe they’re riskier options.)
Just as in 2009, there’s no shortage of skeptics regarding the future of renewables — and just as in 2009, the skeptics appear to be making a good bet. (Though, it’s a perverse one, since the winners will suffer from a climate-ravaged world along with the rest of us). The challenge ahead is enormous, and the odds are undoubtedly against us. Yet, I expect that when I reach my retirement party — presumably in 2050, gazing back over the past 30 years — I’ll be surprised by the history that’s unfolded. By 2050, I believe the world will be mostly decarbonized, and therefore mostly electrified.
So, how do we get there from here?
Where we’re headed
Here’s a back of the envelope illustration of the amount of wind and solar energy we’d need to decarbonize the current US electrical grid:
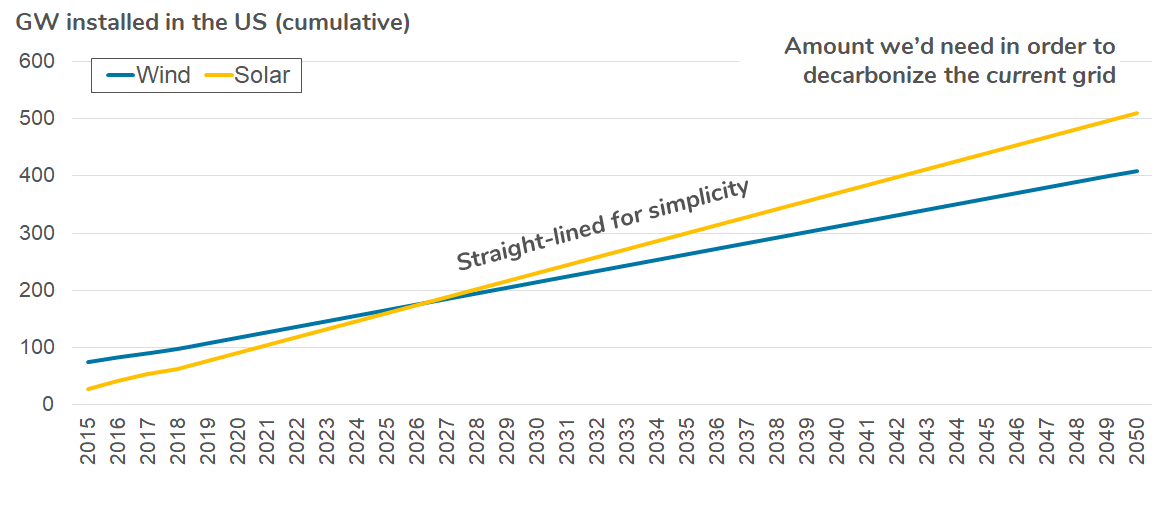
That’s a lot of renewables (and this analysis assumes we’ll keep most of our existing hydro and nuclear power plants up and running, which is nowhere near guaranteed). But of course, decarbonizing the current grid isn’t anywhere close to sufficient for society-wide decarbonization. We’ll also need zero-carbon energy to satisfy the roughly 80% of energy demand in the US that isn’t currently powered by electricity. At this point, the clearest pathway for decarbonizing those end uses is to begin with renewables, and end with either electrification, or with hydrogen that’s produced via clean electricity. (More on hydrogen below….)
Meeting this demand creates an additional challenge: Electrifying most end-uses means, at a minimum, doubling the amount of clean electricity generation we need to deploy.
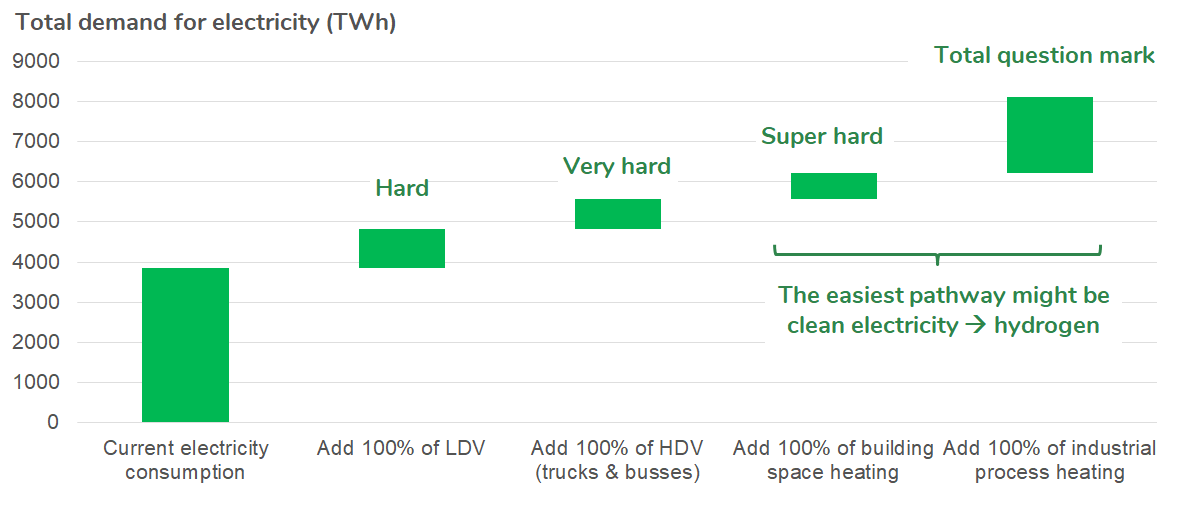
That means we’re going to need about 10 times as much wind and solar capacity as we have today — installing on average 3 times as much renewable power per year as we’ve added to the grid in each of the past few years.
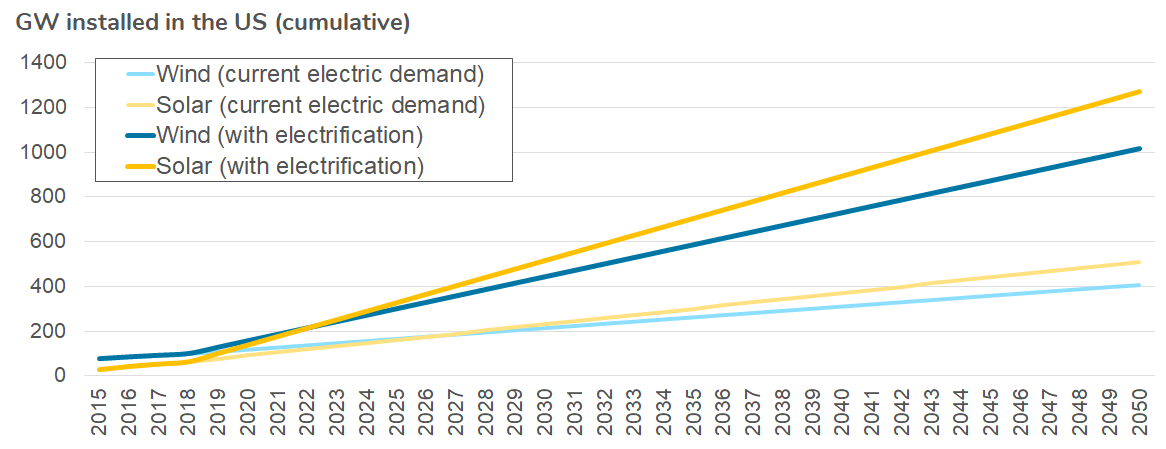
In sum, if we pursue this extreme decarbonization & electrification pathway:
We’ll need to triple our rate of wind and solar deployment, and keep up that pace for 30 years straight. Simultaneously, we’ll need to electrify almost all of our cars and trucks, which means that the electrical grid will need to deliver about 50% more energy.
We’ll also need to figure out a way to employ wind and solar power to heat our homes, buildings, and industrial processes. That means converting a portion of our heating systems to run on electricity, which will tax the electrical grid even further. Very likely, it also entails turning a whole lot of wind and solar power into hydrogen, while converting a whole lot of furnaces, boilers, and industrial systems so that they can burn hydrogen instead of fossil fuel.
We need to accomplish all of the above at a cost that’s socially and politically permissible. And we need to do it without compromising the reliability of energy supply, which today is north of 99.8%.
That’s no easy feat, but I believe it’s achievable. The tremendous advances made by the wind and solar industries over the past decade have given us most of the tools we need.
Hence, the rest of this series is about the rest of the tools we’ll need to decarbonize our energy system, but don’t yet have in our grasp. These tools are still in their infancy — or, in some cases, have yet to be born — but I believe at this point we can already see the outlines of their maturation.
Here are those outlines, distilled into four major categories:
1. Two distinct storage technology revolutions
- One storage revolution for vehicles, which calls for batteries that are about twice as dense and half as expensive as standard electric vehicle batteries today, and are able to charge comfortably in about 10 minutes
- One storage revolution for grid balancing, which requires a way to store massive quantities of energy from season to season at an order of magnitude lower cost than today’s batteries
2. Offshore wind that’s cheaper and capable of being installed further from shore, or an alternative clean energy generation technology that can power densely populated coastal cities through dark winter nights
3. Clean heat for buildings & industrial processes, most likely including some combination of the following:
- Major progress on the clean hydrogen supply chain — especially electrolysis (the process of turning electricity and water into hydrogen and oxygen)
- New techniques for employing electricity or hydrogen to generate industrial process heat
- Cheaper, more efficient, cold-weather-capable electric heat pumps
4. A suite of technology to secure the reliability of a more dynamic, distributed, and ever more essential electrical grid
In the articles to follow, I’ll delve into each of those categories in turn.
But first, let’s talk about hydrogen
One of the first books I read when I was becoming interested in energy issues was “The Hydrogen Economy”, by Jeremy Rifkin, first published in 2002. The book argued that hydrogen had a major role to play in the global economy as a sort of “clean energy medium of exchange”. Since then, the core arguments in favor of that thesis haven’t really changed, but most of the arguments against have grown stronger. However, I still believe hydrogen has a major role to play in the coming decades of the energy transition. Let me explain.
I’ll start with the main arguments in favor of hydrogen:
1. Hydrogen can be produced via clean electricity. Today, the vast majority of commercial hydrogen, which is used for a number of industrial purposes, is produced via the carbon-intensive steam reformation of methane. But the technology to produce hydrogen from electricity and water — electrolysis — is well established. So is the inverse technology — the fuel cell — which turns hydrogen back into water and electricity. So, if we clean up power generation, then the full ‘hydrogen cycle’ would be clean, as well.
2. Today, we transport and store the majority of our energy in the form of oil & natural gas, in large pipelines and tanks. Hydrogen could, hypothetically — with some adjustments — make use of the majority of the infrastructure designed for gas (and perhaps some designed for oil). If, on the other hand , we opt for electricity as our sole “energy medium of exchange”, we’ll need to at least double the energy carrying capacity of the electrical grid (see above), while all of those pipelines will be left to rust. Even more critically, we are still unable to cost-effectively store massive amounts of electrical energy for periods of weeks to months — I’ll discuss this challenge in detail in a subsequent article. But, we could hypothetically store plenty of energy in the form of hydrogen, in existing oil and gas tanks, or in naturally-occurring underground caverns.
3. Vehicles present very different energy storage problems than the grid — problems of density and flow. In terms of volume, the liquid form of hydrogen isn’t as ‘energy-dense’ as oil-based fuels (which are truly remarkable energy carriers, despite their shortcomings). But hydrogen holds about four times as much energy in the same space as a Lithium-ion battery — which is the most energy-dense, rechargeable battery technology that’s commercially available today. Also, hydrogen flows just as quickly as oil, making the process of ‘refueling’ a vehicle with hydrogen comparable to the process of filling up at the gasoline pump — unlike batteries which take 30–40 minutes to fill up even at today’s so-called “fast” charging stations.
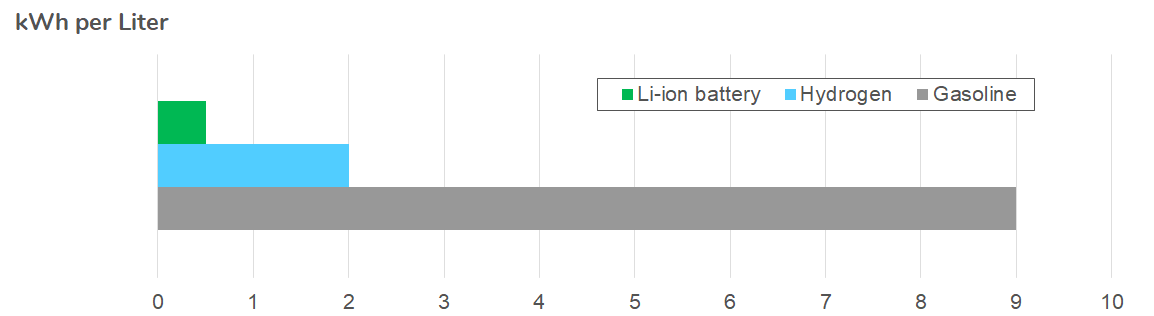
1. The infrastructure transition weakness: It’s easy to say “we can employ existing gas infrastructure for hydrogen”, but it’s not so easy to accomplish in practice. That infrastructure is still being used — and will be for decades, even in an optimistic scenario — by natural gas. Unlike the electrical grid, which is indifferent to the source of the electrons it carries, gas pipelines are built for purpose. While they could hypothetically be retrofitted to accept 100% hydrogen, those retrofits can’t occur while hydrocarbons are still flowing through the tubes.
So, hydrogen needs to be transitioned into its eventual home, but in the meantime it’s not the most welcome guest. Currently, most countries only permit up to 2% of the energy content of the natural gas network to be made up of hydrogen. The most permissive country on that front — the Netherlands — permits up to 4%. From pipe and joint materials, to pressure settings, to end-user tolerances for gas ‘impurity’, there are too many variables to enable a smooth transition from one fuel source to another in our gas infrastructure.
2. The Betamax versus VHS weakness: Sometimes technologies don’t win because they’re better; they win because they’re first, or because they have better marketing, or because of some other historical accident. And for most major energy consuming activities — i.e. driving cars, heating buildings, and even some industrial processes — electricity is already beginning to gain a foothold.
Hydrogen might hypothetically have advantages over electricity as our go-to energy carrier, if we were starting from scratch. But due to the idiosyncrasies of the real world, electricity is starting to looking more like VHS, and hydrogen looks more like Betamax.
And yet, hydrogen has staked a claim on a number of end-use sectors into which it would be extremely difficult for electricity to encroach. In the sectors that are most difficult to completely electrify — such as heavy duty transport, aviation, space heating, industrial process heating, and primary materials manufacturing — I’m convinced we’ll eventually come to rely on hydrogen. This is the view taken by the Hydrogen Council — a global initiative sponsored by a number of large industrial companies — which has developed a plausible scenario in which hydrogen serves as the carrier for roughly a fifth of total energy demand by 2050.
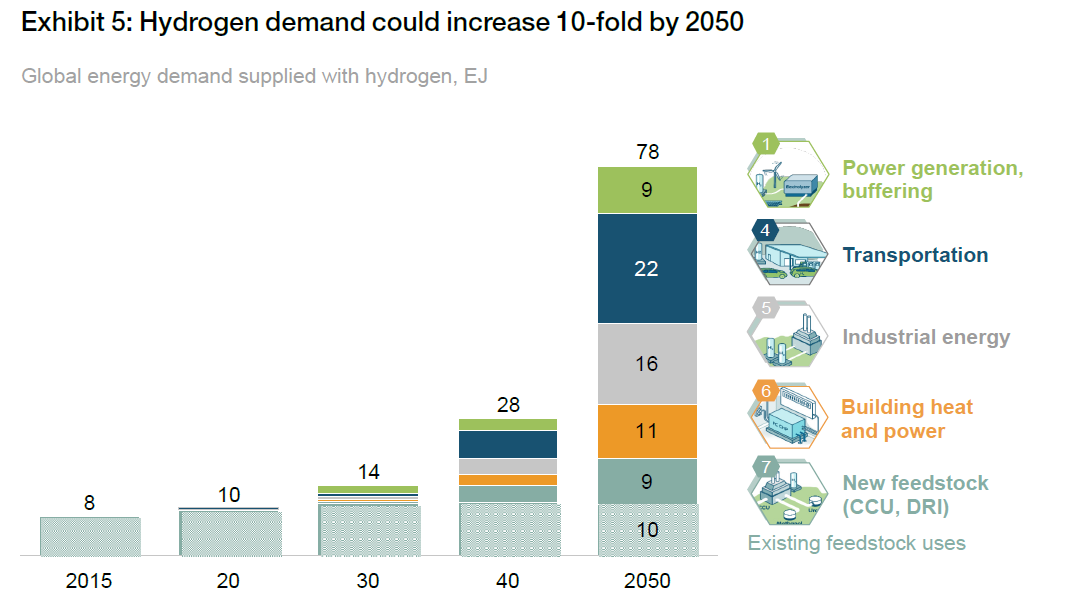
It’s important to note that in this scenario, the majority of hydrogen produced would not be converted back to electricity. The majority would be consumed directly as a fuel for heating, or as a feedstock for primary materials manufacturing. Hence, our focus for the majority of hydrogen R&D efforts ought to be the technology & infrastructure that we’ll need to produce hydrogen, store it, and transport it — rather than the fuel cell technology that converts hydrogen back into electrical energy.
First and foremost among the processes requiring investment is electrolysis. Currently, the cost of hydrogen produced via electrolysis is 10–20 times the cost of producing an equivalent amount of natural gas.
Some of this cost differential is due to the miniscule scale of the electrolysis industry, which barely shipped enough capacity last year to ingest even the output from a single modest wind farm. Economies of scale will almost certainly make a big difference in driving down the cost of the equipment.
In addition to up-front cost, maximizing electrolyzer utilization will also be a critical determinant of overall hydrogen economics. It’s a tricky one, because demand for hydrogen will inevitably surge in the winter heating season. Hence, a robust national system of hydrogen transport and storage will be essential for making sure electrolyzers can run full-tilt during the summer. (“Make hay hydrogen when the sun shines.”)
Lastly, electrolyzer efficiency needs to improve. As discussed above, the current predominant electrolysis technology — proton exchange membranes — are only about 60% efficient. Researchers have been trying to commercialize another technology, solid oxide electrolysis, which is more like 80% efficient, but the up-front cost has been a major impediment.
In sum: Hydrogen is probably going to be a player in the energy transition, but not as the energy ‘medium of exchange’ that was once believed to be its presumptive role. Instead, hydrogen will be mostly employed as a delivery medium for the most difficult to electrify end uses. For other end uses, electricity is now looking like the probable winner.
…Which brings me to energy storage technology, coming up next!
24 March 2020
Climate Action