The Growing Threat: How Climate Change Disrupts the Global Water Cycle
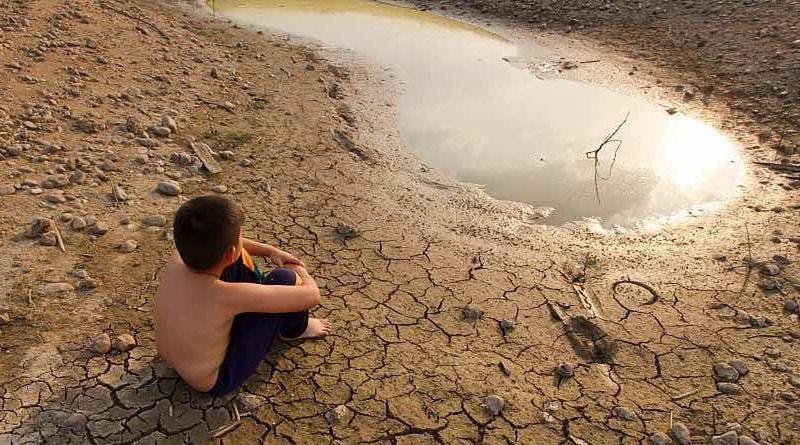
The global climate is changing rapidly, primarily due to human activities that emit greenhouse gases into the atmosphere. One of the most significant impacts of climate change is on the water cycle. Water is intrinsically linked to climate, and even minor changes in climate can have major impacts on water resources.
According to the Intergovernmental Panel on Climate Change (IPCC), "Climate change can alter precipitation patterns, induce extreme weather events, reduce water availability, degrade water quality and increase water temperatures, with detrimental effects on freshwater systems."
Some of the key ways that climate change affects the water cycle and water resources include:
- Altered precipitation patterns and increased rainfall variability
- Melting of glaciers and reduced snow cover
- Sea level rise and saltwater intrusion into groundwater
- Longer and more intense droughts
- Increased frequency and severity of floods
- Changes to groundwater recharge
- Higher water temperatures
- Impacts on water quality and ecosystem health
These impacts on water availability, quality and distribution have widespread implications for humans and ecosystems. Water is essential for drinking, sanitation, agriculture, industry, transportation, energy production, and sustaining natural environments. Thus, climate change impacts on water have broad social, economic, political and environmental consequences across the world.
According to the World Health Organization (WHO), "climate change affects the social and environmental determinants of health – clean air, safe drinking water, sufficient food and secure shelter." Adequate water resources are crucial for reducing poverty, increasing food security, maintaining human health, and supporting sustainable development. As climate change exacerbates water-related risks, vulnerable communities will be disproportionately affected.
Tackling climate change and adapting to its impacts are critical to ensure the long-term sustainability of our water resources. This requires reducing greenhouse gas emissions, improving water management practices, investing in infrastructure and technologies, and enhancing regional cooperation on shared water resources. With proactive and collaborative action, we can increase the climate resilience of water systems around the world.
The Hydrological Cycle
The movement of water on Earth is an intrinsically interconnected process known as the hydrological cycle. This continuous cycle circulates water between the land, ocean, and atmosphere through various pathways:
Evaporation - Solar energy causes water at the surface to turn to water vapor and evaporate into the air. Major sources include oceans, rivers, lakes, soils, plants, and glacial meltwater.
Transpiration - Plants release water vapor to the air through tiny pores in their leaves during photosynthesis. This accounts for about 10% of atmospheric moisture.
Condensation - As moist air rises and cools, water vapor condenses onto microscopic particles in the atmosphere to form clouds.
Precipitation - Moisture in the clouds falls back to Earth in the form of rain, snow, sleet, or hail. On average, about 78% of annual precipitation occurs over oceans.
Infiltration - Precipitation that falls onto land can infiltrate into ground, replenishing soil moisture and aquifers.
Surface Runoff - Excess precipitation flows overland towards streams, rivers, and lakes, eventually draining into oceans.
Groundwater Discharge - Groundwater seeps out to oceans, rivers, springs and wetlands through the subsurface.
This continuous cycling of water is driven by solar energy. As climate change alters temperature, precipitation patterns, and the timing/extent of snow/ice cover, it can fundamentally impact every step of the water cycle. Even small changes can propagate through the entire system. Tracking and understanding these hydrological shifts is crucial for adapting water management to a changing climate.
Impacts of Climate Change on Water
Climate change is intensifying the global water cycle, causing significant shifts in water availability, quality, and extreme events. Some of the major observed and projected impacts on water resources are outlined below.
Melting Glaciers
Glaciers are sensitive indicators of climate change, and global warming over the past century has resulted in unprecedented glacier retreat. According to the IPCC, worldwide glacial mass loss between 2006 and 2015 was "the highest for any decade over the period of observations."
Rising temperatures are melting glaciers in alpine regions, the Arctic, and Antarctic. Over 90% of alpine glaciers worldwide are retreating. Since 1961, glacier volume has declined by 9,625 cubic kilometers, contributing to over 25% of observed sea level rise.
This glacier melt affects water resources in several ways:
- Initially increases meltwater flow as ice melts rapidly
- Reduces natural water storage and alters seasonal availability
- Causes formation of glacial lakes that may suddenly burst, causing catastrophic flooding
- Threatens water supply in glacier-fed rivers used by over a billion people
- Contributes to sea level rise, increasing coastal flooding and saltwater intrusion
Major glacier-fed rivers such as the Indus, Ganges, and Yellow Rivers provide water for drinking, irrigation, and hydropower for hundreds of millions in Asia. Continued glacier retreat will severely impact water security in these regions. Adapting reservoir operations and water infrastructure will be essential to buffer against shifting meltwater patterns.
Changing Precipitation Patterns
Higher global temperatures are disrupting historic precipitation levels and increasing rainfall variability. While total annual precipitation is expected to rise, it will be distributed unevenly across regions. Some areas are projected to see increased rainfall while others experience drying trends.
There have already been significant observed changes in precipitation over land:
- Increased precipitation in eastern North America, northern Europe, northern Asia
- Drying trends in the Mediterranean, southwest Australia, southern Africa
- Increased frequency of intense rainfall events in many regions
According to NASA, a warming atmosphere holds more moisture, intensifying rainfall during storms. Extreme precipitation events will continue to become more common, increasing local flood risk.
Shifting rainfall patterns have major implications for agriculture, ecosystems, flood control, and water storage needs. Better monitoring and climate forecasting will be essential to help communities adapt. Enhancing regional cooperation and water-sharing agreements for transboundary basins impacted by changing precipitation is also critical.
Increased Occurrence of Droughts
Higher temperatures coupled with changing rainfall patterns are increasing drought frequency and severity in many regions. The IPCC states that droughts have become more common in the Mediterranean, southwest United States, southern Africa, and other areas since the 1970s.
During droughts, reduced precipitation and higher rates of evaporation decrease soil moisture, deplete surface water supplies, and lower groundwater tables. This can devastate crops and livestock, reduce hydropower output, disrupt industrial/municipal water supply, and harm aquatic ecosystems.
Even regions projected to see increased annual precipitation may endure longer or more intense intermittent droughts between rainfall events. Hotter temperatures will exacerbate drought impacts by drying out land and water surfaces.
Managing drought risk through early warning systems, water conservation policies, insurance schemes, and emergency relief funding is an increasing priority. Developing resilient agriculture and infrastructure will also help communities endure droughts.
Sea Level Rise
Rising global temperatures are causing sea levels to rise through thermal expansion of ocean water and increased melting of land-based ice sheets and glaciers. Sea levels rose around 20 centimeters in the 20th century and the rate continues to accelerate.
According to the IPCC, mean sea level could rise by up to 1 meter by 2100 if greenhouse gas emissions remain very high. This will Cause permanent coastal flooding and loss of low-lying areas. Small island nations are especially vulnerable, with some at risk of total submersion.
Beyond inundation of coastal zones, sea level rise also pushes saltwater further inland, contaminating estuaries, aquifers, soils and groundwater. Intrusion of saline water into freshwater resources threatens water security of coastal communities and island nations. Preventing saltwater intrusion requires managing pumping from coastal freshwater wells and implementing barriers or injection wells.
Installing desalination plants powered by renewable energy can supplement freshwater resources compromised by saltwater intrusion. But this technology requires substantial infrastructure investment. Poorer island countries face severe water security challenges from sea level rise, highlighting the injustice of climate impacts.
Impacts on Groundwater
Climate change will affect natural groundwater recharge rates. In some regions, increased precipitation intensity may enhance aquifer recharge. But shifts in seasonality of rainfall along with higher evapotranspiration could reduce infiltration in other areas.
Rising sea levels also drive saltwater further into coastal aquifers. In addition, over-extraction of groundwater and land use changes such as urbanization can diminish recharge potential. Depleting groundwater outpaces natural recharge in many major aquifer systems today, including in India, North Africa, and the central U.S.
Sustainably managing both climate change impacts and human demands will be crucial to ensure long-term groundwater security. Natural infrastructure solutions like reforestation, wetland restoration, and reduced soil tillage can improve local recharge capacity. At the same time, improving irrigation efficiency, regulating well pumping, and increasing water recycling can reduce pressure on limited groundwater.
Water Quality Impacts
Climate change degrades surface water and groundwater quality through several mechanisms:
- Higher water temperatures reduce dissolved oxygen levels, harming aquatic life
- Increased severe rainfall washes more pollutants and sediments into waterways
- Droughts concentrate contaminants at lower water flows
- Flooding can damage sanitation infrastructure, spreading pathogens
- Algal blooms thrive in warmer waters, producing toxins
Lower water quality threatens drinking water safety, ecosystem health, and water treatment costs. Waterborne illness outbreaks are expected to become more frequent with climate change impacts.
Adapting will require upgraded water treatment infrastructure, enhanced pollution control, improved sanitation, and increased water quality monitoring. Phytoremediation and constructed wetlands can help filter contaminants naturally. Community-level water quality data collection efforts are also emerging globally.
Impacts on Ecosystems and Biodiversity
Changing precipitation patterns, melting glaciers, increased water temperatures, drought, and declining water quality – all undermine the health of aquatic and water-dependent ecosystems.
With global warming, many plant and animal species migrate poleward or upward seeking cooler habitats. But species in isolated alpine or poleward ecosystems have nowhere to move. Extinction risk thus grows for cold-climate species like pikas, harlequin frogs, and meltwater stoneflies.
Rising water temperatures also shift aquatic species distributions, allowing invasion of exotic species. Droughts shrink wetland breeding habitat for amphibians and waterfowl. And glacial meltwater flooding scours streambeds, damaging spawning areas.
Conserving key freshwater ecosystems through protected areas buffers against biodiversity loss. Maintaining habitat connectivity facilitates species migration induced by climate shifts. Reducing other stressors like land-use change and pollution builds ecosystem resilience to endure climate impacts.
Water and Food Security
The agricultural sector accounts for about 70% of global water withdrawals. With a growing population, food production must expand substantially in coming decades to meet increasing food demand. But climate change poses a major threat to water resources essential for agriculture.
Water for Agriculture
Irrigated agriculture relies on reliable water supplies to cultivate crops and sustain livestock. In addition, rainfed agriculture depends on consistent precipitation patterns. But climate change is increasing rainfall variability, heat waves, and drought in many regions – undermining stable crop yields.
More frequent and lengthy droughts can devastate agricultural productivity. The IPCC states that some African countries could see yield losses up to 50% by 2020 from droughts. Increased drought in Asia would threaten rice and wheat crops that feed billions.
At the same time, higher year-round temperatures boost evapotranspiration by crops and soils. Plants thus need more water to thrive, just as supplies become less reliable. Rising heat also reduces productivity of livestock and poultry.
Adapting irrigation infrastructure and practices to climate change is imperative to protect food security. Upgrading irrigation efficiency, expanding water storage and conveyance capacity, and switching to less thirsty crops can help farmers endure droughts.
Impacts on Food Production
Overall, climate change is expected to slow growth in global food production. Yields of major cereal crops like maize and wheat begin declining with just 1°C of warming. Temperature rise greater than 3°C could cause precipitous crop failures and yield declines.
Sea level rise and saltwater intrusion into deltas also threaten major rice cultivation regions across Asia. Coastal flooding and erosion worsens with more intense storms, reducing fertile agricultural land.
Fisheries that provide essential nutrition for billions are increasingly disrupted by water warming, ocean acidification, deoxygenation, and intensified storms. Climate impacts across land and sea thus jeopardize food supplies worldwide.
Food price spikes related to droughts and extreme weather are already becoming more common, exacerbating malnutrition globally. Sustaining food security requires both adapting agriculture to climate change and reducing greenhouse gas emissions to minimize temperature rise.
Managing Water Resources
Careful management of water resources is vital for ensuring sufficient supply for both agriculture and other uses including drinking, industry, and ecosystems.
Some strategies for balancing agricultural water needs and climate risks include:
- Improving irrigation efficiency through techniques like drip irrigation, precision application, and deficit irrigation
- Expanding rainwater harvesting infrastructure on farms
- Switching to less water-intensive crops suitable for local climate conditions
- Managing groundwater sustainably through regulated pumping and recharge projects
- Reforestation, reduced tillage and other approaches to enhance soil moisture retention
- Agricultural insurance products that help farmers endure weather-related crop losses
- Regional integration of water allocation policies and infrastructure
- Improved climate monitoring and seasonal forecasting to anticipate droughts and adapt plantings
Investing in both natural and built water infrastructure is essential to increase resilience. But reducing greenhouse gas emissions remains imperative to limit unprecedented global temperature rise and associated agricultural water risks.
Extreme Weather Events
More intense and frequent extreme weather events related to climate change pose major risks to water security and quality. Key concerns include floods and stronger storms.
Floods
A warming climate accelerates the global hydrological cycle, increasing extreme precipitation that causes severe flooding. Intense rainfall overwhelms drainage infrastructure and waterways, inundating communities and land.
The IPCC states that flood frequency and intensity is rising across most world regions. Damaging floods resulted in over 100,000 deaths and affected 2.3 billion people between 1995-2015, with costs exceeding $660 billion. Flood risk continues escalating with climate change.
In addition to intense rain events, accelerated melting of glaciers and ice sheets is creating dangerous glacial lake outburst floods. Sudden breaching of these expanding high-altitude lakes unleashes catastrophic flooding downstream. Lives and infrastructure are being lost from the Andes to the Himalayas due to the phenomenon.
Adapting to increased flooding requires both natural and engineered solutions:
- Expanding wetland areas to absorb floodwaters
- Restoring watershed vegetation to slow runoff
- Improving storm drainage capacity in flood-prone settlements
- Upgrading flood defenses like levees and seawalls
- Establishing floodplain zoning and relocating communities out of risk zones
- Implementing early warning systems and emergency procedures
- Expanding insurance coverage to help communities bounce back
Balancing gray and green infrastructure investments can enhance flood resilience while also improving water capture and quality co-benefits.
Storms
Warming oceans provide more energy to tropical storms, increasing their intensity. Climate change has already expanded the latitudes storms reach as temperatures rise further from the equator.
The proportion of strong hurricanes (Category 4-5) has grown over the past four decades along with ocean heat uptake. Rapid intensification of storms is also becoming more likely.
Stronger storm surges worsened by sea level rise cause severe coastal flooding and erosion damage. Wind damage and heavy precipitation from intense storms also clobber water infrastructure like drainage systems, reservoirs, treatment plants and pipes.
Stormwater pollution surges flood waterways, carrying nitrogen from fertilizers, sewage, toxics and debris into ecosystems. Cholera and other waterborne diseases spread if sanitation systems fail. Drinking water becomes unsafe without power for filtration and pumping.
Improving resilience involves modifying building codes and infrastructure design to handle climate-aggravated storm impacts. Expanding natural buffers like mangroves and wetlands helps shield coastlines. Early warning systems, disaster planning, and post-storm funding are also crucial for climate-proofing storm-prone regions.
Adaptation and Solutions
Alongside urgent greenhouse gas emission reductions, water resource management must adapt to climate change risks. Various strategies and technologies exist to improve climate resilience and security of water supplies.
Water Conservation
Water conservation encompasses all measures aimed at using water more efficiently to reduce overall demand. With climate change straining water availability in many regions, conservation grows increasingly vital.
Some effective urban water conservation policies and practices include:
- Installing low-flow fixtures such as toilets, faucets, and showerheads
- Replacing inefficient appliances like washing machines and dishwashers with high-efficiency models
- Adopting drought-tolerant native landscaping that minimizes irrigation needs
- Using precision irrigation systems attuned to weather conditions
- Designing water-smart public spaces and buildings to recycle water onsite
- Installing smart water meters to identify leaks and shape consumer behavior through conservation pricing
- Encouraging individual actions like taking shorter showers, fully loading the dishwasher, and washing full loads of laundry
Many utilities have achieved substantial demand reductions through these combined approaches. For example, San Antonio’s water conservation efforts cut per capita use from 225 gallons per day in the 1980s down to 134 gallons by 2016.
In the agricultural sector, upgrading to efficient micro-irrigation systems like drip and sprinklers can reduce water use 30% or more compared to conventional flood irrigation. Deficit irrigation, alignment with crop needs, and other improved farm practices also help optimize agricultural water productivity.
Water Reuse and Recycling
Water recycling captures wastewater or stormwater, treats it, and then uses it again either for the same purpose or a different one. For example, recycling can take sewage effluent and make it suitable for irrigation, industrial processes, or even drinking, after advanced treatment.
Water reuse helps communities, businesses and agriculture derive more benefit from limited supplies. It can also reduce discharge of pollutants into freshwater bodies.
Some examples of water reuse include:
- Using treated wastewater in agricultural and landscape irrigation
- Augmenting drinking water supplies with purified recycled water
- Capturing stormwater runoff for non-potable uses
- Recirculating water through multiple cycles at an industrial plant
- Flushing toilets with greywater from sinks, showers, etc.
In Israel, recycled wastewater supplies 50% of irrigation needs. Singapore aims to raise non-potable recycled water to 55% by 2060. As climate change intensifies droughts, reuse and recycling will only grow more crucial globally.
Desalination
Desalination is a technology that removes salts and minerals from seawater or brackish water to provide fresh, potable water. As climate change and human activities strain limited freshwater resources in many coastal areas, desalination is increasingly being utilized.
There are two main desalination processes:
- Reverse osmosis (RO) filters pressurized saline water through polymer membranes that contain microscopic pores to block passage of salt ions. This accounts for about 60% of installed desalination capacity.
- Multi-stage flash distillation (MSF) heats saline water to produce water vapor, which condenses on cold surfaces as freshwater and is collected. About 40% of capacity uses MSF or variations.
Desalination plants provide freshwater in arid regions like Saudi Arabia, United Arab Emirates, Israel, and Australia. However, energy demands are still high. Improving efficiency and shifting to renewable energy can reduce environmental impacts. Costs are also falling as membrane technology improves.
Smaller, modular desalination systems powered sustainably have potential to provide freshwater resilience, especially on remote islands. But desalination should be part of a diversified strategy along with conservation, efficiency, and water reuse.
Watershed and Wetland Restoration
Natural landscapes like forests, floodplains and wetlands regulate water flow, filter contaminants, and sustain biodiversity. But development, agriculture and pollution have degraded many watersheds.
Restoring natural infrastructure through projects like reforestation, meadow conservation, and wetland construction can replenish water resources and resilience.
Watershed restoration re-establishes vegetation, reconnects rivers to floodplains, removes dams or embankments, revives drainage channels, and improves farm tillage practices. Healthier watersheds recharge groundwater, reduce flooding, and improve water quality.
Wetland restoration involves replanting native vegetation and rewetting dried-out marsh areas. Wetlands soak up floodwaters, filter pollutants, sequester carbon, and provide critical wildlife habitat.
Protecting and reviving natural watershed infrastructure maximizes services ecosystems provide for water security. Nature-based solutions are often more sustainable and cost-effective than solely relying on built infrastructure.
Improved Irrigation Techniques
Flood irrigation across countless farms wastes enormous volumes of water through leakage, evaporation and runoff. Upgrading traditional flood methods to efficient pressurized irrigation can save substantial water as climate change makes conservation imperative.
Drip irrigation systems apply water directly to crop roots through perforated tubes or drippers. This minimizes losses while precisely meeting crop needs. Drip irrigation can reduce water use 30-70% compared to conventional methods.
Sprinkler irrigation sprays water out through mechanical or pressurized nozzles mounted on pipes. Types like center-pivot or wheel-line sprinklers allow accurate irrigation control and 30-50% water savings.
Subsurface irrigation pipes or tubes buried underground minimize exposure to wind. Trees and vines can be irrigated via buried drip systems. Vegetables may use shallow buried tubes with porous walls that gradually exude water into soil.
Precision irrigation utilizes soil moisture sensors, weather data, and plant water needs to guide timing and amounts. Automating irrigation allows remote control and optimizing for real-time conditions.
Upgrading traditional irrigation brings substantial climate adaptation, water security and food production benefits. However, the shift requires considerable investment and training for farmers. Financial and technical assistance is essential to enable widespread adoption.
Drought Monitoring and Early Warning
Timely drought monitoring and early warning helps communities minimize impacts proactively. Rather than simply responding to dire crises, early warning enables adaptive steps before conditions deteriorate catastrophically.
Components of effective drought early warning include:
- Hydrological monitoring – Measuring rainfall, streamflow, reservoir levels, groundwater tables, and snowpack to detect deficits
- Remote sensing data – Satellite imagery tracks vegetation health and land surface moisture to identify drought emergence
- Drought indices – Mathematical indicators that quantify intensity based on rainfall shortages, soil moisture, etc.
- Impact assessments – Documenting effects on crops, livestock, water supply, wildfires, ecosystems
- Seasonal climate forecasting – Predictions of precipitation and temperature used to project drought likelihood
- Information dissemination – Communicating drought status alerts to government agencies, stakeholders, media and the public
By monitoring relevant indicators and delivering actionable information to communities, early warning provides lead time to implement water conservation, crop insurance, livestock protection, awareness campaigns, and emergency response planning before droughts become calamitous.
Flood Management
Several strategies can help communities become more resilient to increased flooding driven by climate change.
Natural flood management utilizes landscape features to absorb and slow down floodwaters. Projects include wetland restoration, reforestation, reconnecting rivers to floodplains, and rural land practices to increase water infiltration.
Improved storm drainage expands conveyance capacity and adds detention ponds or tanks to reduce urban flood risk. Adding green infrastructure like rain gardens and bioswales further absorbs storm runoff.
Upgraded flood defenses such as levees, seawalls and floodgates help protect settlements located in vulnerable floodplains and coastal zones, if designed accounting for projected climate impacts.
Floodplain regulation limits construction in high-risk areas while preserving floodplains as green space. Removing structures already located on floodplains also reduces exposure.
Flood insurance helps cushion financial blows to people and businesses suffering losses. However, it should be priced appropriately to reflect growing climate risks.
Early warning systems allow advance preparations like temporary barriers and preemptive evacuations ahead of major inundation events, saving lives.
Integrated flood management coordinates infrastructure upgrades, disaster prevention, community preparedness, insurance, and early warning. But increased floods also highlight the importance of global climate action to stabilize the disrupted hydrological cycle.
Infrastructure and Technology Investments
Adapting water systems to climate change often requires major infrastructure upgrades and deployment of new technologies.
Some examples include:
- Expanding water storage capacity with larger reservoirs, tanks and aquifer storage to capture shifting precipitation patterns
- Conveyance infrastructure like canals, pipes and pumping stations to move water from areas of relative surplus to those facing increased scarcity
- Seawalls and levees to protect coastal freshwater supplies and communities against storms and rising seas
- Micro-irrigation systems like drip and sprinklers to conserve agricultural water
- Advanced water treatment through membranes, UV, advanced oxidation to enable reuse of alternate sources
- Desalination plants to create new freshwater from seawater or brackish sources
- Flood drainage works including conveyance tunnels, detention basins and pump stations
- Renewable energy systems to sustainably power energy-intensive water infrastructure
- Hydro-meteorological monitoring technologies that provide data to guide adaptive water management
Upgrading infrastructure and expanding supply requires major capital investment. But it is essential to bridge the growing climate adaptation gap in water management. Pursuing lowest-cost and no-regrets solutions first can help maximize benefits.
Integrated Water Resources Management
Traditionally, water management has been fragmented across siloed sectors like drinking supply, irrigation, hydropower, etc. But effectively adapting water systems to climate change requires holistic and coordinated management across uses.
Integrated Water Resources Management (IWRM) enables coordinated, sustainable water management by:
- Managing across sectors like agriculture, energy, environment, municipalities, industry
- Harmonizing groundwater, surface water, water quality, and ecosystem management
- Linking policy domains like land use, pollution control, conservation
- Bridging geographical scales from local watersheds to regional transboundary basins
- Building multistakeholder collaboration across communities, governments and the private sector
Key strategies encompassed in IWRM include:
- Monitoring hydrological systems
- Allocating water based on efficiency and priorities
- Pollution regulation
- Participatory decision-making
- Combining built infrastructure, policy, and institutional improvements
IWRM enhances climate resilience by leveraging synergies across sectors and users, allocating limited water flexibly as conditions change, and sustaining ecosystems. Adaptive, integrated management across scales is essential to navigate climate uncertainties.
Public Awareness and Education
Expanding public awareness and education on climate change threats to water resources empowers communities to take action. Engaged citizens can drive policy changes, conserve water, and build local resilience.
Some strategies for raising public awareness include:
- Highlighting climate impacts on local water resources through media stories and campaigns
- Promoting water conservation practices for homes, businesses and agriculture
- Providing educational curricula and resources on water and climate in schools
- Training farmers and agricultural extension agents on climate risks and adaptive techniques
- Communicating drought and flood monitoring information through bulletins and text alerts
- Warning vulnerable communities about climate health hazards like poor water quality or vector-borne disease
- Encouraging citizen climate and water quality monitoring efforts
- Organizing participatory scenario exercises and discussions
- Building social media campaigns, especially targeting youth
Informed and engaged communities that understand climate threats are better equipped to drive solutions. Expanding climate change education and participation thus enables locally rooted resilience.
The Role of Climate Action
While climate change adaptation in water management grows increasingly urgent, aggressive mitigation to reduce greenhouse gas emissions remains essential to control the scale of temperature rise and associated water impacts.
Mitigation Efforts
The more planet-warming pollution can be reduced today, the less catastrophic climate change impacts will become in the long-run. Ambitious mitigation action is thus crucial to avoid surpassing dangerous temperature thresholds that would devastate water resources, ecosystems and communities globally.
Some key mitigation strategies related to the water sector include:
- Phasing out coal power and rapidly upscaling wind, solar, geothermal and other renewable energy generation to cut emissions from the electric sector
- Dramatically improving building and appliance efficiency to reduce electricity and heating fuel consumption
- Accelerating the transition to electric vehicles, efficient mass transit, and smarter urban planning to achieve low-carbon transportation
- Implementing methane capture systems to control emissions from wastewater treatment
- Restoring forests, wetlands and other carbon-sequestering landscapes
- Encouraging low-carbon diets with less water-intensive meat consumption
- Curbing food loss and waste across the supply chain to optimize resource use
Every country and economic sector must pursue aggressive mitigation actions to reach net-zero emissions by mid-century. Without such collective action, climate change will continue disrupting water systems and lives across the planet.
The Paris Agreement
In 2015, nations adopted the landmark Paris Agreement under the United Nations Framework Convention on Climate Change (UNFCCC), committing to limit global warming to well below 2°C above pre-industrial levels, and to pursue efforts to limit warming to 1.5°C.
Over 190 countries have signed on, establishing Nationally Determined Contributions (NDCs) to cut emissions. However, current NDCs remain insufficient to meet targets – deeper reductions are urgently needed. The Paris Agreement also calls for climate finance to help developing nations adapt and transition toward sustainable, climate-resilient water and other infrastructure.
The Paris Agreement signals strong multilateral commitment to finally curb greenhouse gas emissions after decades of delays. But countries must continually strengthen targets and policies to achieve its ambitious goals. Ongoing civil society pressure and intra-national actions by states, cities and businesses also remain critical to bend the global emissions curve.
Sustainable Development Goals
Adopted by all UN member states in 2015, the 2030 Agenda for Sustainable Development establishes 17 Sustainable Development Goals (SDGs) to end poverty, reduce inequality, protect the environment and foster prosperity.
Clean water and sanitation are highlighted in SDG 6. Other goals also incorporate key targets related to water resources, such as:
- Increase water-use efficiency and ensure freshwater supplies (SDG 6)
- Implement integrated water resources management (SDG 6)
- Protect and restore water-related ecosystems (SDG 6)
- Expand water harvesting and storage capacity (SDG 6)
- Reduce deaths from water-related disasters (SDG 11)
- Strengthen climate resilience and adaptive capacity (SDG 13)
Pursuing the SDGs drives progress on interlinked water, climate, poverty reduction, and environmental objectives. But achieving the bold vision requires mobilizing trillions in public and private sector investment in sustainable infrastructure globally.
The Water-Energy-Food Nexus
Water availability, energy production, and food security are closely interdependent. This “nexus” must be jointly considered to enable sustainable development and navigate trade-offs.
For example, irrigation requires pumping groundwater or diverting rivers, consuming energy. Thermoelectric power generation utilizes water for cooling. Hydroelectric dams provide electricity but alter river flow for ecosystems and agriculture. Biofuel and shale gas production can pollute and deplete shared water sources.
Meanwhile, rising energy demands and climate change constrain available water for farming. And fertilizer and pumping for irrigation consume energy while boosting food production.
Pursuing narrow objectives in any one sector often spurs unintended impacts in the others. Policies and investments must holistically optimize trade-offs and synergies across the water-energy-food nexus. For instance, drip-irrigated solar pumping can simultaneously enhance water efficiency, energy access and agricultural yields.
Conclusion
Climate change poses grave risks to water security worldwide. However, societies still have time to adapt and build resilience to coming disruptions in collaboration with the natural environment. There are no silver bullet solutions, but implementing a mosaic of conservation, infrastructure, technology, policy, and governance strategies can effectively improve climate risk management.
Innovations like precision irrigation, water reuse, green infrastructure, integrated resource planning, and early warning systems highlight the capacity for adaptation. However, rapid reduction of greenhouse gas emissions remains imperative to prevent catastrophic global warming beyond adaptation limits. The fate of water resources ultimately hinges on urgent climate action.
With informed, inclusive policies and public participation, we can transition toward water stewardship that is flexible, integrated and just. While climate change brings unprecedented challenges, it also provides opportunity to correct unsustainable use, protect ecosystems, and cooperatively manage shared waters. With wise foresight and courage, we can cultivate resilient water systems to sustain flourishing communities and environments despite climate uncertainty. Our collective future depends on living in balance with water and climate.